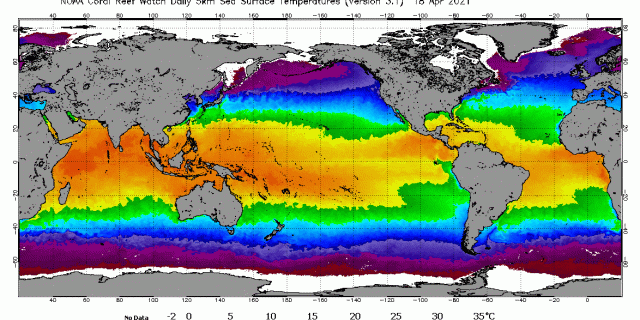
Tom Tamarkin and Bud Bromley
June 20, 2024
A common misconception propagated by the media and alternative energy companies today is that man contributes to the increasing concentration of carbon dioxide (CO2) in the atmosphere. This is simply not true. Atmospheric CO2 concentration is controlled by fundamental laws of physics and chemistry. Man could increase his current release of carbon dioxide from the burning of natural gas and fossil fuels by a factor of ten and the net total amount of CO2 in the atmosphere would be roughly the same as it is today. Conversely, if man eliminated his CO2 releases, the atmospheric concentration would remain the same as it is today. CO2 is naturally maintained in dynamic equilibrium with the environment.
The total amount of CO2 in the atmosphere is independent of biogenic and anthropogenic CO2 generation and primarily dependent on temperature gradients on the surface of the oceans. The Earth’s atmosphere contains a trace amount of CO2 at 0.04% in a gaseous mixture of predominately nitrogen, oxygen, and argon. All life forms on Earth are carbon based. Plants acquire their carbon from gaseous CO2 in the atmosphere and convert it to plant cells through photosynthesis. Mammals acquire their carbon by eating plants or other animals.
Biogenic CO2 is produced naturally by animals, plants, bacteria and the decomposition of organic matter. Some CO2 is deeply sequestered for millennia in earth or in water as stone (aragonite, limestone, calcium carbonate, CaCO3) and some is continuously recycling between atmosphere, ocean surface, plants, soil, and sea life, like plankton and algae. When deeply as stone, CO2 is re-emitted released into the atmosphere and ocean by very high heat burning the rock, for example volcanic activity, most of which occurs in deep ocean waters.
Anthropogenic CO2 is produced by burning hydrocarbon fuels, land use changes, and the production of cement. The ocean contains about 50 times more CO2 gas than the atmosphere. Ocean surface alone contains about 100 times more CO2 gas than emitted by human use of fossil fuels. Concentration of CO2 gas and dissolved ionic forms of carbon increase significantly with ocean depth, density and the colder temperature of deep ocean. Dense, cold, deep water holds far more CO2 gas and other carbon forms than surface water. Surface ocean holds far more CO2 gas than atmosphere. CO2 gas molecules in the atmosphere are continuously colliding with earth’s surface and being absorbed. Since the ocean is about 70% of earth surface, most CO2 is absorbed into ocean surface. Simultaneously, earth’s surface, primarily ocean, is emitting CO2 into the atmosphere. The ratio of absorption versus emission is known as Henry’s Law constant. The Henry’s Law constant is a ratio that varies primarily with the temperature of the ocean surface, but also the temperature of water everywhere in plants, soil, animals, raindrops, etc. There is a Henry’s Law constant for all gas and liquid combinations and CO2 gas and seawater is only one example combination. Ocean surface temperature is continuously changing by day and night, clouds, seasons, currents, winds, latitude, etc. Colder water increases the ratio of CO2 gas absorption; warmer water increases the ratio of CO2 gas emission; temperature of the surface interface between the gas and liquid changes the Henry’s Law constant.
Seasons also cause large changes in the CO2 concentration in the air due to seasonal changes in absorption and emission of CO2 by plants and sea life such as plankton and algae. The amount of these seasonal natural changes dwarf human CO2 gas emissions. For example, in the Northern Hemisphere’s springtime earth’s surface is rapidly warmed by the sun and thus CO2 gas concentration in air steeply increases to its seasonal maximum as CO2 gas emission from warmer ocean and soil exceeds CO2 gas absorption. Then, coming into the Northern Hemisphere’s summer and autumn, plant life on land and ocean is growing vigorously by absorbing CO2 gas from air to use in photosynthesis and growth. So, like clockwork, every year in summer and autumn there is a rapid decline in atmospheric CO2 gas concentration as plant life reaches maximum growth. But, simultaneously in the same summer and autumn season, ocean and land surface have also warmed, and thus natural CO2 gas emissions are increasing relative to natural CO2 absorptions, partially offsetting the rapid increase in seasonal absorption of CO2 gas by land and aquatic plants. Every year there is a repeating shark’s teeth pattern of rapid increase followed by rapid decrease in net atmospheric CO2 gas concentration as CO2 gas emitted by all sources cycles back and forth continuously with CO2 absorbed by all CO2 sinks.
Notice this cycling of CO2 gas described above is natural and is not controlled by humans! The amount of cycling CO2 is much larger than the amount of CO2 emitted by humans and the human CO2 is immediately mixed with the natural CO2. Estimates vary, but deep ocean contains about 38 to 40 times more carbon than ocean surface and about 50 times more than atmosphere. Notice in these graphics that the cycling of both absorption and emission in and out of ocean (~92 gigatons and ~90 gigatons respectively) are over 10 times more than the ~5.5 gigatons of CO2 emitted from fossil fuels. The CO2 from fossil fuels immediately becomes part of the much larger natural carbon cycle.
Estimates vary of the carbon cycle, but CO2 from human use of fossil fuels is negligible compared to the natural cycle.
Discussion of Applicability and Operation of Henry’s Law and Raoult’s Law in the Atmospheric Biospheric Exchange:
In his classic and very detailed textbook on Aquatic Chemistry, Dr. Werner Stumm covers many different aspects of Henry’s Law, including CO2 in water and seawater.
“The physicochemical significance of Henry’s Law is this: there is a linear relationship between the activity of a volatile species in the liquid phase and its activity in the gas phase.”…”Henry’s Law is an observational result for a two-phase equilibrium A(l) = A(g) under dilute solution conditions and for low pressures… “The distribution of gas molecules between the gas phase and the water phase depends on the Henry’s Law equilibrium distribution. In the case of CO2, SO2 and NH3, the dissolution equilibrium is pH dependent because the species in the water phase – CO2(aq), H2CO3, SO2, H2O(aq), NH3(aq) – undergo acid base reactions.”
(Stumm, Werner, 1996. Aquatic Chemistry, p213. https://archive.org/details/aquaticchemistry0000stum/page/192/mode/2up)
Henry’s Law is the fundamental technology underlying the multibillion dollar per year scientific instrument industry of gas chromatography, which is the crucial technology for separation, identification, quality and process control of virtually all chemical compounds in almost all industries, including EPA, NOAA, etc. and environmental laboratories.
Here is a recent reference for Henry’s Law in water. Compilation of Henry’s law constants (version 5.0.0) for water as solvent By Rolf Sander, PhD. Air Chemistry Department, Max Planck Institute of Chemistry, P.O. Box 3060, 55020 Mainz, Germany. Published: 6 October 2023. https://henrys-law.org
“Abstract. Many atmospheric chemicals occur in the gas phase as well as in liquid cloud droplets and aerosol particles. Therefore, it is necessary to understand their distribution between the phases. According to Henry’s law, the equilibrium ratio between the abundances in the gas phase and in the aqueous phase is constant for a dilute solution. Henry’s law constants of trace gases of potential importance in environmental chemistry have been collected and converted into a uniform format. The compilation contains 46,434 values of Henry’s law constants for 10,173 species, collected from 995 references.” It is available on the internet at https://henrys-law.org
Ammonia (NH3) and references on page 10,915. Carbon dioxide (CO2) and references begin on page 11,190
Henry’s Law does not apply to the reaction products when there is an irreversible or permanent chemical reaction. Dr. Sander explains in his paper in section 2.5, copied below:
“2.5 Effective Henry’s law solubility constants. Hs,eff The Henry’s law constants mentioned so far describe the equilibrium between a chemical species in the gas phase and exactly the same species in the aqueous phase. This type is called the “intrinsic” Henry’s law constant. Once transferred, some species react in the aqueous phase. If these chemical reactions are fast and result in irreversible destruction, Henry’s law cannot be applied. If, however, the species enters a fast equilibrium in the aqueous phase, it is possible to define an “effective” Henry’s law constant Hs,eff, using a “total concentration” ctot. Depending on the chemical class, there are different ways to define such a total concentration.”
The graphic further down below is from Werner Stumm. It illustrates the cyclical, reversible hydration reaction of CO2 and water.
Carbonic acid (H2CO3) is a very minor (only ~0.3% of the CO2) transitory ionic form, almost impossible to measure because it is so transitory in the forward and reverse reactions. H2CO3 exists in water only as ionic species. CO2 hydrates in seawater to form about 90% bicarbonate ion (HCO3–). HCO3– and H2CO3 are reversible by local temperature and chemical conditions to reform aqueous CO2 gas. This subject is covered in more and more detail in the aquatic chemistry textbook by Werner Stumm referenced above.
You can read about CO2 and Henry’s Law with many methods on the following webpage by the U.S. National Institute of Standards and Technology (NIST.) https://webbook.nist.gov/cgi/cbook.cgi?ID=C124389&Units=SI&Mask=10#Solubility
Discussion of Henry’s Law and Raoult’s Law with respect to CO2 interactions in the Earth’s CO2 cycle:
Raoult’s Law and Henry’s Law are two fundamental concepts in chemistry and physical chemistry respectively that describe the behavior of liquid solutions. Both laws help us understand the interactions between the solvent and the solute in a solution, but they differ in their applications and underlying principles. Raoult’s law can be derived from Henry’s law if an ideal solution and an ideal gas are assumed.
Raoult’s Law
Raoult’s Law states that the vapor pressure of a solute molecule above a solution is equal to the arithmetic product of the vapor pressure of the pure solute at the same temperature times the mole fraction of the solute present in the solution. This can be represented by a straight line on a graph of vapor pressure vs. mole fraction. Raoult’s law applies to ideal solutions in higher concentrations, where the interactions between the solvent and solute molecules are negligible. Raoult’s Law is useful for predicting the vapor pressure of a solution and is often used in the calculation of the boiling point elevation and freezing point depression of a solution. Raoult’s Law typically describes ideal behavior of pure and high concentration solvents in dynamic equilibrium with the partial vapor pressure of that solvent in the gas phase above the solution.
Henry’s Law
Henry’s Law, on the other hand, applies to solubility or concentration of non-ideal, very dilute gases within liquid matrices, both ideal and non-ideal. A Henry’s Law constant is a proportional ratio observed in thousands of experiments with thousands of combinations of gases and liquids. A Henry’s Law constant for a specific surface temperature the partition ratio of (a) divided by (b), where (a) is the partial pressure or concentration of a specified gas in a liquid matrix, and (b) is the partial pressure or concentration of that same gas in the gas phase matrix above the liquid surface. Henry’s Law is useful for predicting the ratio of gas emission vs gas absorption from and to a liquid surface at a specified temperature. Henry’s Law states that the solubility of a dilute gas in a liquid is proportional to the partial pressure of the dilute gas in contact with the liquid. This can be represented by a straight line on a graph of solubility vs. partial pressure. Practically, the partial pressure of a dilute gas in a liquid is extremely difficult or impossible to precisely measure. Alternatively, Henry’s Law can also be stated as the ratio of (a) the concentration of a dilute gas dissolved in a liquid divided by (b) the concentration of the same gas above and in contact with the liquid. Henry’s law applies to the solubility of a specified dilute and unreacted gas in a specified liquid at a specified liquid surface temperature. Henry’s Law is used to calculate gas concentrations in blood and other biological fluids and tissues by controlling the partial pressure of the inhaled gas, for example an anesthetic gas. Henry’s Law describes non-ideal behavior in that part of the gas may react with the liquid matrix and in that case the reacted part is excluded from the Henry’s Law ratio, and also the ratio applies to low concentrations (approaching infinite dilution).
Summarizing and simply said: total CO2 is a very low concentration, i.e., trace gas in the atmosphere, but it is essential to all life. CO2 gas in ocean is also very low concentration. The human contribution to this trace amount is trivially negligible and being continuously mixed with the much large total amounts in air and ocean. Addition to the atmosphere of the relatively (compared to the total) small amounts of CO2 gas from fossil fuels is rapidly and continuously absorbed by the natural environment until the natural Henry’s Law ratio is restored. Attempts to sequester or remove CO2 from the atmosphere result in rapid replacement of that CO2 by the environment until the natural Henry’s Law ratio is restored. CO2 gas in air is in dynamic equilibrium in the environment. Like almost all perturbations in nature, the rate and scale of restoration of a perturbed equilibrium is proportional to the rate of change and relative mass of the perturbation (Le Chatelier’s principle and the Law of Mass Action.)
Key Differences
The main differences between Raoult’s Law and Henry’s Law are:
Application: Raoult’s Law applies to the vapor pressure of an ideal gas above an ideal liquid solution, and high concentrations. Henry’s Law applies to solubility of a low concentration gas in a liquid solution, ideal or not, so long as the gas is dilute, and any products of reaction of the gas with the liquid (or any component in the liquid) are not included in the ratio. Both Raoult’s Law and Henry’s Law applies only to unreacted solutes.
Raoult’s Law applies to concentrated, pure, or dilute vapors (i.e., evaporated liquids or dissolved and/or evaporated solids). Henry’s Law applies to a specified dilute gas within a gas phase matrix transitioning to and from that same specified dilute gas within a liquid phase matrix. The rates of these two Henry’s phase-state changes are not parallel because of the large pressure and density difference between a liquid phase matrix and a gas phase matrix.
In Raoult’s Law the ratio is (a) divided by (b) where (a) is the amount of the solute in the solvent liquid, and (b) is the amount of solvent.
In Henry’s Law, the ratio is (a) divided by (b), where (a) is the amount of solute gas (e.g., CO2) in the liquid mixture (e.g., seawater), and (b) is the amount of the same solute gas in the mixture of gases (e.g., air) above the liquid.
Principles: Raoult’s Law describes the discovery by experimental observations that the vapor pressure of a solute molecule above a liquid solution surface is equal to the vapor pressure of that pure solute at the same temperature times the molar fraction of that solute in the liquid solution; doubling the concentration of solute A in the solution results in doubling the vapor pressure of A above the solution surface. Measuring the vapor pressure of the solute above the surface predicts the amount of solute in the liquid. Or, measuring the vapor pressure of a solute molecule above a solution predicts the concentration of the solute molecule in the liquid.
Henry’s Law describes the discovery by experimental observations that the solubility of any gas in any liquid is directly proportional to the partial pressure of that gas which is in contact with the liquid surface; doubling the concentration of the gas in the gas phase above and in contact with the liquid will double the solubility of that gas in the liquid. An increase in partial pressure of a specific gas above the liquid surface will result in an equal increase in the partial pressure (i.e., solubility) of the same gas within the liquid surface. And vice versa, when partial pressure of the gas decreases above the liquid surface, such as by CO2 sequestration or surface winds, then the gas will be emitted from the liquid surface until the Henry’s ratio is restored for the specified gas/liquid combination at the specified surface temperature. Time to absorption gases into liquids is longer than time to emit gases from liquid.
Henry’s Law expressed analogously to Raoult’s Law: the quantity of a gas dissolved in a liquid is proportional to the quantity of the gas in the gas phase in contact with the liquid.
Derivation: Henry’s law constant is a proportionality coefficient describing the distribution of a gas between the liquid phase and the gas phase above and in contact with the liquid surface. Henry’s coefficient can be defined as solubility or as volatility or in dimensionless form. However, great care must be taken with units when converting one form to the other to avoid confusion and mistakes. The temperature dependence of Henry’s Law constants has been derived. (1)
In climatology with atmospheric gases such as CO2 it is convenient to use the dimensionless form of Henry’s law constant because the defacto gold standard for atmospheric gases, such as CO2 reported by the Global Monitoring Laboratory at NOAA-Scripps Mauna Loa, has routinely for many decades measured micromoles of CO2 per mole of freeze-dried air, which is identical to ppm and reported as ppm. Conveniently, in the dimensionless version the molar units cancel out in the division arithmetic. (Note: ppmv results in significant errors since water and water vapor have been frozen out of the air samples before measurement which requires changing the units (units are Moles per kilogram mass instead of Moles per liter air volume) due to high variability of volume with changes in humidity and water content in air. For example, according to NOAA GML calculations for the year 2020, the difference in the measured amount of CO2 in their freeze-dried air samples vs the measured amount of CO2 in their wet air samples was 14 times the average annual increase in CO2 concentration.
Scope: Raoult’s Law is limited to ideal solutions and the vapor pressure above the solution, while Henry’s Law applies to a wider range of physical chemical systems, including non-ideal solutions and gases, and mixed phases such as biological fluids. A specified gas solute has a unique Henry’s Law constant for a specified liquid solvent at a specified temperature. Henry’s Law constants are easily looked up in reference books and online, having been determined experimentally thousands of times as referenced in the works of Dr. Rolf Sander. Most importantly, and this is commonly misunderstood, Henry’s Law only applies to the unreacted gas solute; that is, Henry’s Law does not apply to any reaction products resulting from a solute gas reacting (i.e., a change of molecular structure) with the liquid solvent, nor does Henry’s Law apply to the products of the gas solute resulting from reactions with any other solutes in the liquid matrix. To be clear, Henry’s Law does not apply to the carbonate, carbonic acid and bi-carbonate reactions and their subsequent reaction products such as calcium carbonate which occur in seawater after CO2 gas has hydrated and become an ionic reaction product. However, these ionic reactions subsequent to CO2 hydration are rapidly (seconds) reversible, producing again unreacted aqueous CO2 gas in seawater, which may potentially emit CO2 gas from seawater surface, and again must be included in the Henry’s Law ratio. The hydration kinetics constant of unreacted aqueous CO2 gas is ~ 15 kcal/mol at 25°C only ~1 kcal/mol less than ~ 16 kcal/mol at 20-25°C to dehydrate H2CO3 to unreacted aqueous CO2 gas (Stumm, W. 1996. Aquatic Chemistry. https://archive.org/details/aquaticchemistry0000stum/page/192/mode/2up). This sensitive reaction may be activated forward or reversed variously by:
- By sunlight changes at ocean surface, due to various causes,
- by warming the seawater, e.g., via warm upwelling convection of other water currents due to various causes,
- surface seawater oversaturated with aqueous CO2gas, e.g., via an upwelling current oversaturated with CO2 gas from deep ocean, or
- changing seawater alkalinity, pH, e.g., by dilution with rainfall (which is lower pH, lower alkalinity than seawater)
- changing seawater salinity, e.g., by rainfall
- Increasing surface winds and/or sea surface agitation/waves which increases sea surface area thus increasing depletion of sea surface of aqueous CO2 gas causing the bicarbonate and carbonic acid reaction to reverse and produce more aqueous CO2 gas in the seawater surface. The following graphic from the Werner Stumm (reference below) may be useful:
- The unreacted CO2gas dissolved in the ocean water comprises only about 1% of the initial CO2 reactant amount absorbed into ocean surface, dependent dominantly on surface temperature, while the carbonate, carbonic acid, bi-carbonate ionic reaction products comprise about 99%.
- The dimensionless Henry’s Law coefficient is the ratio of the concentration of the gas in the liquid phase divided by concentration of the gas in the gas phase [(cl)/(cg)], that is, moles of unreacted, non-ionic, non-hydrated CO2gas per kilogram of ocean water divided by the moles of unreacted CO2 gas per kilogram of air above the ocean water surface.
- The Henry’s Law ratio is a constant for any given combination of solute gas and solvent liquid at a given surface temperature because the diffusion rate of any gas is the inverse of the square root of the molecular weight of the gas; the is Graham’s Law. The molecular weight of a gas is a constant.
Conclusion: Raoult’s Law and Henry’s Law are two important concepts in chemistry and physical chemistry describing the behavior of solutions and gases. While both laws are useful for calculating the properties of volatile solutes and liquid solutions, they differ in their applications and underlying principles.
Raoult’s Law is used to calculate the vapor pressure of solutes above ideal liquid solutions at a given temperature or to predict the amount of a solute mixed in a solvent by measuring the vapor pressure of the solvent above the liquid at a given temperature. Henry’s Law is used to calculate the solubility rate of a gas in a liquid, or to calculate the ratio of the amount of a gas in a liquid versus the amount of the same gas in above the liquid at a given surface temperature.
Raoult’s Law is silent with regard to reaction of the gas, liquid or solid solute with the liquid solvent. Henry’s Law does not apply to the reaction products of gases and liquids, nor does it apply to concentrated gases, nor to non-gas phase solutes. Henry’s Law defines the ratio of a continuous, simultaneous bidirectional gas exchange across a gas/liquid surface interface; the Henry’s Law partition ratio is dynamic, being mediated primarily by temperature of the gas/liquid surface interface.
- Compilation of Henry’s law constants (version 5.0.0) for water as solvent By Rolf Sander, PhD. Air Chemistry Department, Max Planck Institute of Chemistry, P.O. Box 3060, 55020 Mainz, Germany. Published: 6 October 2023. https://henrys-law.org
- U.S. National Institute of Standards and Technology (NIST.) https://webbook.nist.gov/cgi/cbook.cgi?ID=C124389&Units=SI&Mask=10#Solubility
- Stumm, Werner, 1996. Aquatic Chemistry, https://archive.org/details/aquaticchemistry0000stum/page/192/mode/2up)
- Original 1802 published work by William Henry